Neutron stars and their “violent deaths”: gravitational waves.
Neutron stars are the end result of massive stars’ explosive deaths. These stars, with masses 15 to 20 times that of our sun, run out of fuel and collapse. This collapse forms a black hole, sending out gravitational waves that travel through space-time.
Studying these waves helps us understand neutron stars better. They also reveal the secrets of these stars’ explosive endings. Gravitational waves can be detected from as far as 50 million light-years away, making them key to modern astrophysics.
There’s a growing interest in finding gravitational waves from neutron star collisions. These waves are a major breakthrough in astronomy. They let us uncover the universe’s mysteries, including the violent deaths of stars and other cosmic events.
In this article, we’ll dive into neutron stars, their explosive ends, and how gravitational waves help us grasp the universe.
Understanding Neutron Stars
The definition of neutron stars shows they form when a massive star runs out of fuel. This collapse creates an incredibly dense object. It has a mass up to 1.5 times the Sun’s, packed into a space about 20 kilometers (12.5 miles) wide.
Neutron stars have amazing physical traits. A sugar cube of their material weighs about 1 trillion kilograms (1 billion tons) on Earth. Their surface gravity is 2 billion times stronger than Earth’s. Their cores are mostly neutrons, showing unique states of matter.
Neutron stars have incredibly strong magnetic fields. Magnetars, a special type, have fields 1,000 times stronger. For example, SGR 1806-20 released more energy in one-tenth of a second than the Sun in 100,000 years.
Pulsars, a type of neutron star, emit pulses of radiation. These pulses happen in intervals from milliseconds to seconds. The fastest pulsar, PSR J1748-244ad, spins at 716 times per second. Our galaxy has about 2,000 neutron stars, with 1 billion in total.
Characteristic | Value |
---|---|
Mass | Up to 1.5 times that of the Sun |
Diameter | Approximately 20 kilometers (12.5 miles) |
Surface Gravity | About 2 billion times that of Earth |
Sugar Cube Weight | Approximately 1 trillion kilograms (1 billion tons) |
Magnetic Field Strength | Trillions of times stronger than Earth |
Fastest Rotation Speed | 716 rotations per second |
Known Neutron Stars | Approximately 2,000 in the Milky Way |
Estimated Total Neutron Stars | About 1 billion in the Milky Way |
These characteristics of neutron stars show the extreme conditions they face. They reveal the universe’s violent nature. Studying them helps us understand physics and the life cycles of massive stars, leading to new discoveries.
What Causes the Death of Neutron Stars?
Neutron stars die because they can’t fight off gravity. When a massive star runs out of fuel, it can’t push back against gravity. This causes a huge explosion called a supernova.
For stars that are too heavy, a more extreme event happens. It’s called a collapsar. This leads to the creation of a black hole, surrounded by a disk of material.
When a neutron star implodes, its outer layers blast off into space. This creates huge waves that travel through the universe. These waves show how violent and complex the death of neutron stars can be.
Characteristic | Detail |
---|---|
Diameter | Approximately 12 miles (16 kilometers) |
Density | Over 72,000 times denser than the Sun |
Surface Temperature | Averages about 1.8 million degrees Fahrenheit |
Mass Range for Formation | Between 10 and 25 solar masses |
Gravitational Wave Events | Detections confirm binary neutron star mergers, such as event GW170817 |
Escape Velocity | Exceeds half the speed of light |
Rotation | Can rotate several hundred times per second, e.g., PSR J1748−2446ad at 716 RPM |
The death of neutron stars shows how complex and dynamic the universe is. Each explosion adds to the universe’s beauty. It keeps astrophysicists around the world fascinated.
The Role of Gravitational Waves in Astronomy
Gravitational waves in astronomy are a major breakthrough. They offer new views on the universe. These waves, made by big objects moving, change how we see the cosmos.
Their discovery is key, not just because we can see them. They tell us a lot about huge events like when stars merge or black holes crash.
The first time we saw a merger with gravitational waves was on August 17, 2017. It was a big moment. It showed us how these waves help us understand neutron stars.
Neutron stars are very dense and small. They are about 12 miles wide. Even a little bit of their material can be as heavy as Mount Everest.
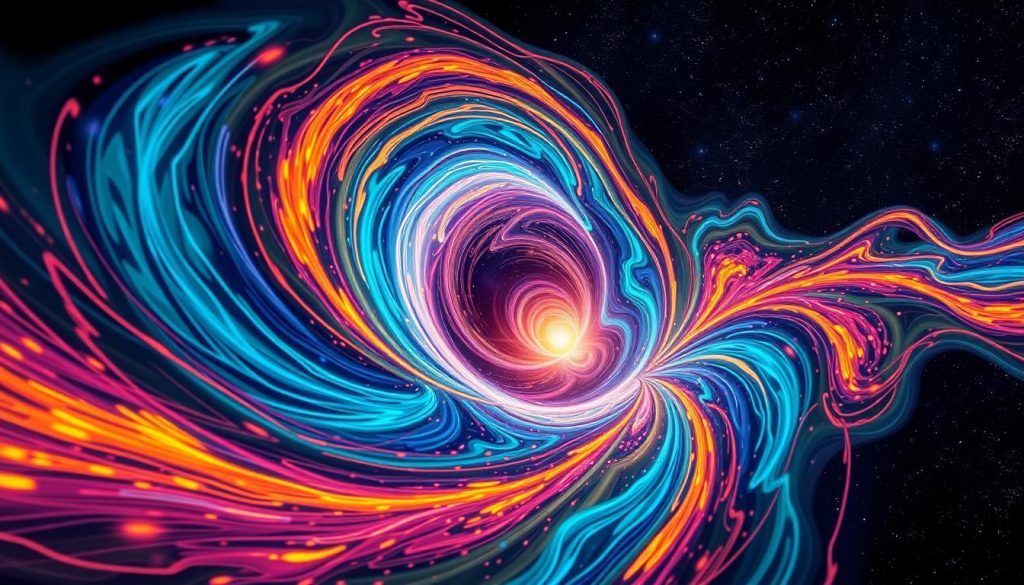
Gravitational waves and optical observations work together. They give us a better story of what happens in space. For example, LIGO detectors help figure out the masses of merging objects.
This helps us know if a new object is a neutron star or a black hole. It also helps us understand how heavy elements in the universe are made.
Gravitational waves are found across many frequencies. Scientists are working to make them more sensitive. This will help us learn more about the universe’s past.
New technologies like the Einstein Telescope and Laser Interferometer Space Antenna will help us. They promise to reveal more about the universe, showing why gravitational waves are so important.
The Formation of Gravitational Waves
Gravitational waves come from extreme events in the universe. They happen when massive objects accelerate, especially during big interactions. For example, when neutron stars merge, they send out waves that we can detect on Earth.
These waves also come from other sources. Neutron stars that spin fast, called pulsars, create them. The collapse of massive stars into black holes is another source. The strength and frequency of these waves depend on the objects’ mass, distance, and speed.
Recent studies suggest that the explosive deaths of massive stars could make very strong waves. These waves might be seen from millions of light-years away. Learning about gravitational waves helps us understand the universe better and how to study these cosmic events.
Source of Gravitational Waves | Characteristics | Example Events |
---|---|---|
Neutron Star Collision | Extreme acceleration, detectable on Earth | GW170817 |
Rapidly Rotating Neutron Stars (Pulsars) | Steady emission, less intense | Detected as periodic signals |
Collapse of Massive Stars | Significant energy release, generates strong waves | Potentially observed from collapsars |
Collapsars: The Explosive End of Massive Stars
The universe is full of wonders, and collapsars are among the most fascinating. These are the explosive ends of massive stars. Stars that are 15 to 20 times the mass of our Sun go through a big change when they run out of fuel. They collapse under their own gravity, leading to a collapsar explosion.
When a collapsar explodes, it happens in two stages. First, it implodes quickly, then it bursts out with energy. Sometimes, this creates a black hole. The leftover star material forms an accretion disk around the black hole. This disk can release a lot of energy and create gravitational waves that travel through space.
Collapsars are important for making the universe richer in heavy elements. When these stars explode, they spread materials that help form new stars and planets. Also, collapsars are linked to long-duration gamma-ray bursts (GRBs), which are much more powerful than regular supernovae. These events happen about 1 to 10 times per galaxy every billion years.
Studying collapsars helps us learn more about the universe. New technology, especially in space telescopes, has improved our understanding of collapsars and their explosions. By studying these events, we gain insights into how stars live and die.
Property | Details |
---|---|
Mass Range | 15 to 20 times the mass of the Sun |
End Phases | C[ollapse to a black hole or supernova] |
Energy Output | Significantly higher than typical supernovae |
Occurrence Rate | 1 to 10 per galaxy per billion years |
Associated Phenomena | Gamma-Ray Bursts (GRBs) |
Detecting Gravitational Waves
Detecting gravitational waves changed astrophysics forever. The Laser Interferometer Gravitational-Wave Observatory (LIGO) led this change. It uses laser beams to measure tiny changes in distance caused by gravitational waves.
The recent discovery of GW230529 shows LIGO’s power. It found a possible neutron star and an unknown object 650 million light-years away. The analysis suggests the smaller object is a neutron star, weighing between 1.2 and 2.0 times our sun.
Gravitational wave observations have made nearly 200 measurements of compact-object masses. Only one other event, GW190814, likely involved a mass-gap object. This progress comes from the work of over 1,600 scientists.
The LIGO-Virgo-KAGRA observing run started in May 2023 and will last until February 2025. This collaboration aims to detect more events. LIGO’s new technology, the quantum “squeezer,” can detect waves from 140 megaparsecs away.
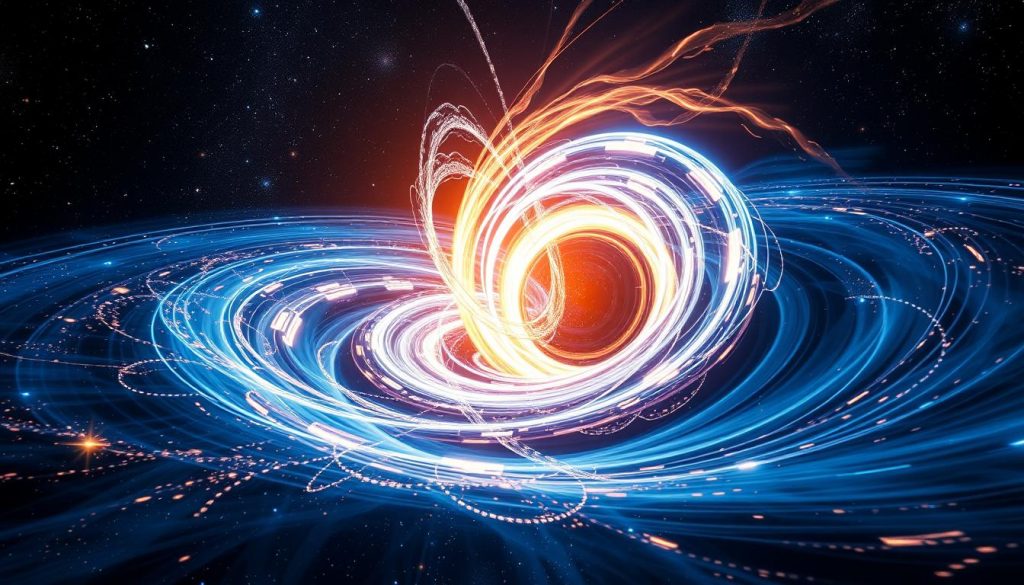
Observatory | Members | Institution Count | Regions/Countries |
---|---|---|---|
LIGO | 1,600 | 2 | USA |
Virgo | 880 | 152 | 17 European Countries |
KAGRA | 400+ | 128 | 17 Countries/Regions |
Since LIGO’s first success in September 2015, there have been nearly weekly gravitational wave discoveries. As technology gets better, we’ll learn more about the universe. This includes secrets about neutron stars.
How Gravitational Waves Were First Detected
The first detection of gravitational waves was a big deal in astrophysics. On September 14, 2015, scientists at LIGO confirmed Einstein’s prediction from nearly a century ago. They found ripples in spacetime from two black holes merging, 1.3 billion light-years away.
This breakthrough was huge. It proved Einstein right and opened doors to new discoveries in astronomy. The laser interferometer at LIGO could spot tiny changes in spacetime, smaller than a proton’s size.
During this event, two black holes with 30 times the Sun’s mass collided. They released energy equal to three solar masses in just a fraction of a second. This success brought excitement and many awards, including the Nobel Prize in 2017.
After that, LIGO kept making new discoveries. For example, on August 17, 2017, it detected gravitational waves from neutron star collisions. This showed LIGO’s growing power and its key role in exploring the universe.
The Significance of Neutron Stars in Gravitational Wave Astronomy
Neutron stars are key in gravitational wave astronomy. Their crashes send out strong signals that we can catch. These signals help us learn about their mass and spin, which are vital for understanding the universe.
The merging of neutron stars brings us new insights into dense matter. For example, GW190814 showed us a neutron star merging with a black hole. This event, 800 million light-years away, was a big find in studying neutron stars.
By studying gravitational waves, scientists can link neutron stars to other cosmic events. Events like GW170817 show how gravitational waves and light signals are connected. This helps us see the whole picture of star deaths and the life of compact objects.
Gravitational waves from neutron star mergers happen about once every few hundred years in a galaxy. Thanks to better technology, we can now see these waves from up to 130 million light years away. As tech gets better, we’ll learn more about the universe through these mergers.
The Physics Behind Collapsar-Driven Gravitational Waves
The physics of collapsars is complex. It involves many factors that lead to the creation of gravitational waves. These waves come from the collapse of massive stars into black holes. They are detectable across vast distances in space.
Gravitational waves from collapsars can be as massive as 15 to 20 times the sun’s mass. This gives us a deep look into these cosmic bodies. Scientists are getting better at simulating these waves. They are learning about the frequency and strength of these waves.
Collapsars offer a new way to find gravitational waves. These waves can be detected from about 50 million light-years away. This is less than the distance of waves from black hole mergers. But it still opens up new areas for space observation.
Here’s a quick overview of collapsar-driven gravitational waves:
Aspect | Detail |
---|---|
Mass Magnitude | 15-20 times the mass of the sun |
Detectable Distance | Approximately 50 million light-years |
Origination Source | Non-merger source for gravitational waves |
Current Observational Capabilities | Possible detection with existing facilities |
Simulation Requirements | Around 1 million collapsar simulations needed |
Energy Release | Greater than \(10^{52}\) erg in BdHN I systems |
Learning about collapsars helps us detect these waves better. It also changes how we think about gravitational wave signals. Scientists expect a rich time for studying gravitational waves with collapsars.
The Future of Gravitational Wave Detection
The future of finding gravitational waves is exciting. New technologies and observatory plans are leading the way. Projects like Cosmic Explorer and the Einstein Telescope will help us see more gravitational wave events.
Right now, the LIGO-Virgo network can measure neutron star properties very well. It might take them decades to get enough data, but Cosmic Explorer could do it in just a year. This shows how fast gravitational wave detection is getting better.
Scientists are also using old data and new methods to find gravitational waves. This will help us learn more about neutron stars and their mysteries.
The table below shows what current and future observatories can do:
Observatory | Time to Collect Data for Precision Measurement | Expected Precision |
---|---|---|
LIGO-Virgo | 10-57 years | 1.9-0.7% |
Cosmic Explorer | 1 year | 0.56% or better |
Einstein Telescope | TBD | TBD |
The future of finding gravitational waves is full of promise. With better tools and methods, scientists are ready to uncover the universe’s secrets.
Conclusion
Exploring neutron stars and their violent deaths shows us a world full of astrophysical wonders. These events create intense gravitational waves. They help us understand how stars evolve and open doors to new discoveries in astronomy.
These waves give us a deeper look into neutron stars. They show us how these stars can change our basic understanding of the universe. As scientists improve their tools for detecting these waves, we expect to learn even more about these cosmic bodies.
Neutron stars are fascinating because of their extreme density and unique behaviors. Recent research suggests protons might be more important than we thought. This is shown by how protons and neutrons work together, as studies from MIT explain.
Studying these stars helps us understand the universe better. It shows us the complexity and vastness of the cosmos. This journey into the lives of neutron stars invites us to explore more. It enriches our view of the universe and our place in it.