The galactic habitable zone: where life may thrive in the universe.
The concept of the galactic habitable zone (GHZ) holds immense significance in understanding the potential for life in the universe.
It refers to specific areas within a galaxy where the conditions are ideal for the emergence and evolution of life.
In the case of our Milky Way, research has identified the GHZ as an annulus with an outer radius of about 10 kiloparsecs, avoiding the perilous vicinity of the galactic center, which is marred by excessive supernova activity.
It is this middle ground that nurtures the formation of habitable planets enriched with crucial heavier elements.
By examining these regions, scientists explore a realm that may harbor the rich tapestry of life beyond Earth. The insights gained about the GHZ not only enhance our understanding of astrobiology but also guide future quests in the search for extraterrestrial life.
As we delve deeper into the factors influencing this zone, we uncover a fascinating interplay between star types, metallicity, and environmental threats that shape the possibility of habitability in our galaxy and beyond.
Factors Determining the Galactic Habitable Zone
The formation of life in the universe hinges on several critical factors of GHZ that shape habitable environments. Our Solar System resides approximately 28,000 light years from the galactic center, providing an ideal distance from potential hazards like gamma rays and cosmic radiation. Such radiation poses threats that can adversely impact life formation, making proximity a crucial consideration.
The metallicity of stars plays a significant role, as only metal-rich stars tend to host rocky planets. A minimum threshold of heavy elements is essential to create these terrestrial bodies conducive to life. More than 95% of stars in the galaxy are less likely to support planets due to disjointed orbits that do not align with the spiral arms. Hence, finding stars with proper alignments increases the chances of discovering habitable environments.
Star density is another factor that influences the GHZ. Regions with higher stellar densities often encounter gravitational perturbations that could disrupt planetary orbits. In contrast, interstellar matter in the spiral arms promotes star formation but introduces potential dangers for solar systems within these regions. These dynamics create a complex web of conditions necessary for stable life formation.
The following table summarizes the key factors affecting the GHZ:
Factor | Impact on Habitability |
---|---|
Metallicity | Higher metallicity increases the likelihood of rocky planets forming |
Proximity to Galactic Center | Increased radiation exposure may hinder life development |
Star Density | Higher densities can lead to gravitational disruptions |
Stellar Orbital Synchronization | Proper synchronization is crucial for supporting habitable conditions |
Interstellar Matter | Facilitates new star formation but can introduce risks |
Metallicity and Its Importance
Metallicity plays a crucial role in the formation and sustainability of planetary systems. It refers to the abundance of elements that are heavier than hydrogen and helium. A higher metallicity in a galactic region supports the availability of essential elements for life, contributing significantly to galactic chemistry and the formation of terrestrial planets.
Regions near the center of the Milky Way typically exhibit increased metallicity compared to those at the edges of the disk. Observations show that stars hosting extrasolar planets generally have higher metallicity levels, while planets in low-metallicity environments are less likely to develop rocky bodies capable of supporting life. This suggests a critical threshold of metallicity exists for the formation of terrestrial planets, although specific values remain to be confirmed.
Interestingly, supernovae occurrences influence metallicity as well. In regions where lethal supernova events are estimated to happen more frequently, such as the inner Milky Way, the effect on the surrounding materials significantly alters the environmental conditions necessary for life. In contrast, the less active outer areas offer a more stable setting.
Understanding the importance of metallicity helps explain why certain parts of our galaxy are considered more promising for life. These high-metallicity areas create favorable conditions for the necessary elements for life to thrive within planetary systems.
Cosmic Catastrophes: Threats to Life
Cosmic catastrophes such as supernovae and gamma-ray bursts significantly influence the potential for life in the galaxy. The increased density of stars, especially near the galactic center, raises the likelihood of such stellar explosions. As a result, this area poses substantial galactic threats to nearby Earth-like planets. Supernova impact can generate intense radiation and cosmic rays that create inhospitable environments, potentially sterilizing large portions of the galactic habitable zone.
According to research, planets located within 13,000 light years from the galactic center encounter a 95% probability of experiencing lethal long gamma-ray bursts over a 500 million year period. As Earth lies approximately 25,000 light years from this center, it too stands a 50% chance of having suffered effects from such events in the past half billion years. Many early planets faced high risks from gamma-ray bursts, drastically reducing the chances for complex biological life to develop in the universe’s initial 8.8 billion years.
Evidence suggests that the outer regions of the galaxy may offer safer havens for life, where the frequency of supernovae is lower. Yet within the inner galaxy, up to 8,000 light years from the center, the production of habitable planets has promising potential despite the risks posed by these cosmic catastrophes. Such contrasting dynamics highlight the ongoing battle for survival in the vastness of space.
Region in Galactic Habitable Zone | Distance from Galactic Center (light years) | Supernova Risk Probability (500 million years) |
---|---|---|
Inner Galaxy | Up to 8,000 | Higher risk due to frequent supernovae |
Mid Galaxy | 8,000 – 13,000 | Moderate risk |
Outer Galaxy | Beyond 13,000 | Lower risk due to fewer supernovae |
The Role of Stellar Types in Habitable Zones
Different stellar types play a crucial role in determining the habitability of planets orbiting them. The star classification system categorizes stars into several types, primarily O, B, A, F, G, K, and M. Each category comes with distinct characteristics, influencing the environmental conditions of surrounding planets.
F, G, K, and M stars are of particular interest when searching for life-bearing planets. G-type stars, such as our Sun, maintain stable conditions that favor the development of life. Their moderate temperatures and extended lifespans allow for the necessary time for complex life to evolve. In contrast, O and B stars have extremely high luminosities and shorter lifetimes, making them unable to sustain the growth of life as we know it.
The habitable zone (HZ) around these stars varies according to their luminosity, dictating the distance needed for liquid water to exist. Within our Solar System, Earth resides near the inner edge of this habitable zone, while Mars is situated just outside its outer edge. Furthermore, the continuously habitable zone (CHZ) extends this concept, representing the area where liquid water remains feasible throughout the star’s Main Sequence lifetime.
The carbonate-silicate cycle further expands our understanding by indicating that the habitable zone could be pushed farther from the star than earlier assumptions suggested. Interestingly, moons like Europa and Titan, despite being outside the CHZ, may still harbor life in some form.
Stellar Type | Lifespan | Habitability Potential |
---|---|---|
O | 10 million years | Too short for complex life |
B | 10-100 million years | Limited, due to fast burnout |
A | 100 million years | Borderline, some potential |
F | 1-2 billion years | Moderate potential |
G | 10 billion years | High potential, ideal for life |
K | 15-30 billion years | Strong potential for life |
M | 50 billion years | Possibly habitable, low likelihood |
Understanding these factors regarding stellar types and their respective habitable zones provides insight into the conditions required for life. As research continues, the relationship between stellar types and habitability will undoubtedly evolve, leading to further discoveries about potential life in the universe.
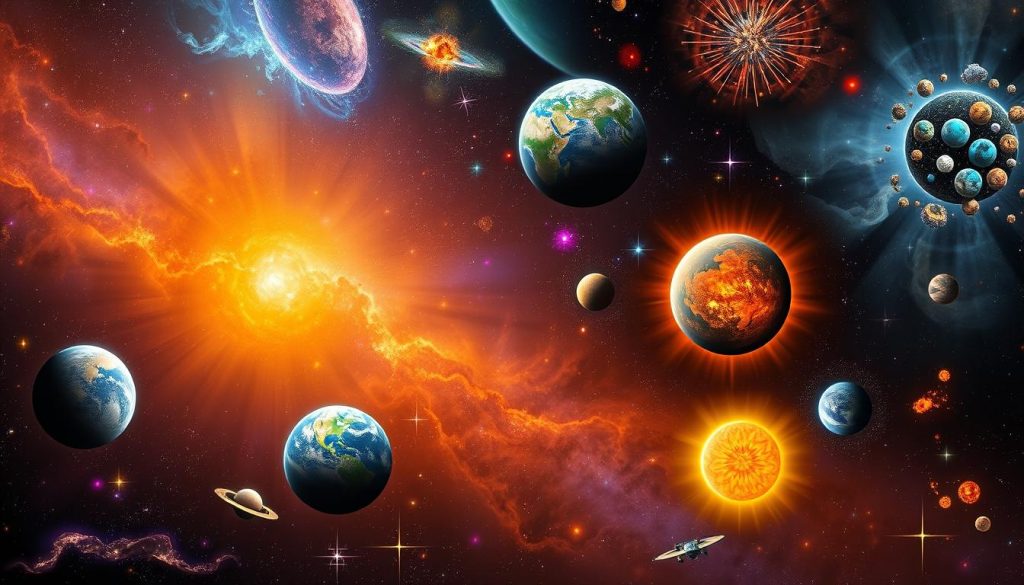
Distribution of Stars in the Galactic Habitable Zone
The distribution of stars within the galactic habitable zone (GHZ) significantly influences the potential for life in the universe. Located approximately 1.5 to 3 kiloparsecs from the Galactic center, this area is theorized to offer conditions conducive to complex life. Within the Milky Way, an estimated 100 billion stars exist, with roughly 10% thought to reside in the GHZ.
The density of stars suitable for hosting habitable planets in the GHZ is projected to be about 100,000 stars per cubic kiloparsec. This dense star distribution aids in gravitational interactions that can foster the formation of stable planetary systems. Closer proximity to other stars may increase opportunities for conditions favorable to life, while also presenting potential hazards.
For regions within the GHZ, an approximate metallicity level of 0.5 solar metallicity enhances the likelihood of forming habitable planets. Complex life is expected to require a stable environment sustained for at least 4 billion years, which aligns with the longevity observed in stars within the GHZ, many having lifespans surpassing 10 billion years.
- Approximately 50% of stars are estimated to host planets.
- G-type stars present a 20% probability of possessing habitable zones compared to lower probabilities for M-types and K-types.
- Optimal distance for life-sustaining conditions often lies between 0.7 to 1.5 astronomical units (AU).
The variations in environmental conditions for habitability significantly depend on factors such as heavy metal content and radiation levels. The degree of influence from nearby cosmic events, like supernovae, also plays a critical role, with such events occurring every 50-100 million years in the GHZ. Understanding the star distribution in this zone will continue to guide researchers in the quest to identify potential life-supporting worlds in our galaxy.
Galactic Bulge vs. Galactic Halo
The galactic bulge and galactic halo represent distinct regions within our galaxy, each exhibiting unique characteristics that significantly influence stellar environments. The galactic bulge, located at the center of the Milky Way, is characterized by a high density of stars, with many dating back over 10 billion years. This region boasts a metallicity that is relatively high compared to the outer reaches of the galaxy, which can be beneficial for planet formation.
In contrast, the galactic halo extends approximately 100,000 light-years from the center and contains older stars with lower metallicity. As a result, the halo is less conducive to the formation of terrestrial planets. The stark differences in stellar environments between these two regions can impact potential habitability.
One notable difference lies in the frequency of cosmic explosions. Studies indicate that events such as supernovae and gamma-ray bursts occur more frequently in the inner regions of the galaxy, putting the galactic bulge at a higher risk of cosmic threats. Such phenomena can dramatically alter local environments, potentially erasing or hindering the development of life.
The contrasting stellar populations between the bulge and halo can also affect the overall habitability of potential planetary systems. Conditions fostered in the galactic bulge may provide a more favorable setting for life compared to the inhospitable nature of the halo.
Feature | Galactic Bulge | Galactic Halo |
---|---|---|
Location | Center of the Milky Way | Surrounding the bulge |
Diameter | Approximately 10,000 light-years | About 100,000 light-years |
Star Age | 10 to 13 billion years | Older stars |
Star Density | 1,000 times greater than the disk | Approximately 50% of the Galaxy’s stars |
Metallicity | Higher metallicity | Lower metallicity |
Risk of Cosmic Events | Higher frequency of supernovae | Lower incidence of cosmic explosions |
Understanding the differences between the galactic bulge and galactic halo plays a crucial role in evaluating potential habitability across various regions of our galaxy. The distinct environments shape the likelihood of life emerging and evolving, leading to intriguing possibilities for future exploration and discoveries.
Impacts of Supernovae on Habitability
Supernovae play a crucial role in shaping the habitability of regions within the galactic habitable zone. These cosmic events not only threaten individual planets but can have far-reaching implications for entire star systems. The aftermath of a supernova explosion generates shockwaves that propel sterilizing radiation across vast distances, impacting neighboring environments. As a result, planets exposed to these supernova impacts experience heightened levels of harmful radiation, which can inhibit the emergence and evolution of life.
The Sun’s formation likely occurred near the galactic center, a region potentially rife with supernova activity. This early environment suggests that the solar system’s relocation to its present orbital radius may carry the lingering effects of past supernovae, possibly contributing to life extinction events. In crowded star-forming regions, the probability of encountering supernovae increases, directly correlating with the escalation of radiation exposure to nearby star systems. Such conditions expose those systems to cosmic hazards that can drastically diminish the chances of developing complex life.
Furthermore, simulations highlight dynamic shifts in the Milky Way’s structural elements, such as the central bar and spiral arms. These changes have significant effects on the transport and habitability of stars, including our Sun. In scenarios where stars undergo trapped migration, they experience dramatic oscillations in their orbital paths. These transitions increase exposure to high levels of star formation and intense gamma-ray bursts, both of which can be detrimental to the viability of potential life forms.
Misaligned star formation regions can compound these risks. Areas outside the galactic habitable zones lack sufficient chemical enrichment, hindering the availability of essential materials for life creation. The presence of supernova explosions in these non-habitable zones further underscores the stark contrasts between regions that foster the origin of life and those that pose existential threats. Engaging in a deeper understanding of supernova impacts informs scientists about the viability of diverse habitable environments across the galaxy.
Factor | Impact of Supernova |
---|---|
Radiation Levels | Increased, leading to potential sterilization of planets |
Star Formation | Heightened risk of encountering gamma-ray bursts and harmful radiation |
Chemical Enrichment | Limited in non-habitable zones, restricting life’s building blocks |
Orbital Dynamics | Dramatic shifts can expose systems to hostile conditions |
Supernova Events | Threat of life extinction across affected regions |
The Significance of Spiral Arms
Spiral arms represent dynamic features within the galactic structure, characterized by high concentrations of gas and dust. These regions are pivotal for star formation, making them vital in the quest to understand where life may emerge. In the Milky Way, the density of stars and molecular clouds in spiral arms plays a significant role in attracting surrounding matter, which influences the formation of planets capable of supporting life.
The Sun, for example, formed approximately 5 kpc from the Galactic center, indicating the importance of positioning within these spiral arms. Its current orbital radius is between 8.5 and 9 kpc, suggesting movement into regions with differing star formation and environmental conditions. The proximity to the galactic center impacts the frequency of encounters with giant molecular clouds (GMCs), raising the chances of exposure to supernovae and gamma-ray bursts (GRBs) that can significantly affect the habitability of planets.
Research underscores that higher metallicity in spiral arms correlates with a greater abundance of essential elements for rocky planet formation. The varied stellar densities and radiation exposure in these regions create complex environments where the potential for life exists, albeit with certain risks. A thorough analysis of the spiral arms within the Milky Way reveals how they impact the characteristics of the Galactic Habitable Zone (GHZ), presenting a balance between resource availability and cosmic hazards.
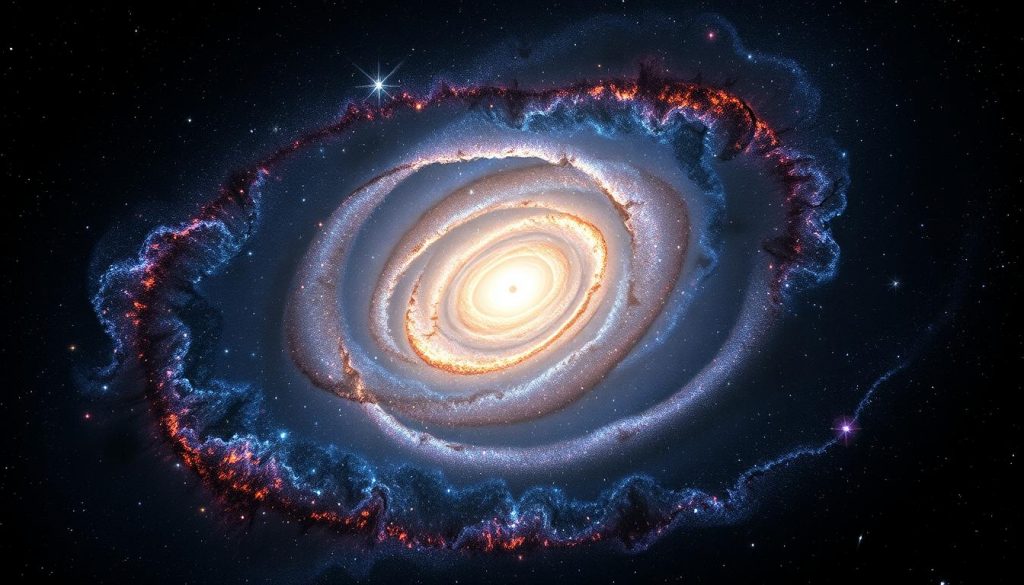
This interplay between star formation and galactic structure highlights why spiral arms deserve focused attention in astrobiological research. Observational data collected via instruments like the Hubble Space Telescope can shed light on patterns of star formation in these areas, providing insights into the conditions necessary for life. In the pursuit of understanding our cosmic neighborhood, recognizing the significant role these structures play becomes increasingly essential. For a deeper exploration of the GHZ and its intricacies, refer to this resource.
Research Findings on Life Beyond Our Solar System
Recent advancements in astrobiology have shed new light on the potential for life beyond our solar system. The investigation of exoplanets within their respective galactic habitable zones reveals increasingly favorable conditions for sustaining life as we know it. Projects like the Kepler mission and the Transiting Exoplanet Survey Satellite (TESS) have identified thousands of these intriguing worlds, offering insights into their potential habitability.
A rocky super-Earth, for instance, would require radioactive isotope abundances approximately 1,000 times higher than Earth’s to maintain long-lived water oceans without exposure to starlight. In contrast, to sustain ethane oceans, it would need only 100 times the isotopes found on our planet. These essential findings highlight the complex factors influencing habitability research.
The concentration of stars in the denser inner regions of the galactic bulge may lead to higher radioisotope abundances due to neutron-star mergers. This suggests an exciting avenue for future studies, as exploring these regions could yield more candidates for potentially habitable exoplanets. The James Webb Space Telescope’s capabilities to detect infrared signatures of internally heated worlds promise to expand the search for habitable conditions beyond traditional boundaries.
Distance and orbital periods of exoplanets, like TOI-715 b, which is located 137 light-years away and is 1.5 times the size of Earth, play a significant role in astrobiology. With an orbital period of just 19 days, its classification of habitable zones—ranging from conservative to optimistic—further emphasizes diverse environments where life may flourish. Meanwhile, the advanced capabilities of the Giant Magellan Telescope and the G-CLEF spectrograph will significantly enhance our ability to analyze the chemical compositions of distant exoplanets.
Exoplanets in the Galactic Habitable Zone
The exploration of exoplanets within the galactic habitable zone has yielded a plethora of exciting discoveries. These worlds exhibit a range of characteristics that may make them suitable contenders for supporting life. Notably, conditions in this zone are considered optimal for the development of habitable planets, unlike the harsher environments closer to the galactic core or the sparse regions of the galaxy.
Among the most compelling findings is the estimated 40 billion Earth-sized planets estimated to orbit within the habitable zones of Sun-like stars and red dwarfs in the Milky Way. Out of these, around 11 billion are believed to be circling Sun-like stars. The proximity of some of these exoplanets, such as Proxima Centauri b—only 4.2 light-years away—fuels curiosity regarding their potential for habitability.
Key factors influencing the habitability of these exoplanets include:
- Size: Earth-sized planets are often more favorable for sustaining life.
- Composition: The presence of liquid water is crucial, and planets that sit in the habitable zone are more likely to have conditions that permit water to exist in liquid form.
- Distance from Their Stars: The right distance allows for stable temperatures and energy inputs.
Research has shown that planets like those found in the TRAPPIST-1 system share several similarities with Earth, making them key candidates in the search for extraterrestrial life. As technology advances, more discoveries are likely to change our understanding of where habitable planets might exist. The insights gained from the current study of exoplanets in the galactic zone will pave the way for future explorations into distant worlds.
Exoplanet | Star Type | Distance (light-years) | Potential for Habitability |
---|---|---|---|
Proxima Centauri b | Red Dwarf | 4.2 | High |
TRAPPIST-1 d | M-type | 39.5 | Medium |
Kepler-186f | Sun-like | 500 | High |
K2-18 b | Red Dwarf | 124 | Medium |
Technological Advances in Identifying Habitable Zones
Recent advancements in technology significantly enhance our ability to explore and understand identifying habitable zones throughout the universe. Instruments like the James Webb Space Telescope and the upcoming Nancy Grace Roman Space Telescope are at the forefront of this revolution, enabling scientists to analyze exoplanet atmospheres more effectively than ever before.
The detection of Earth-like exoplanets poses a unique challenge, as these celestial bodies often shine between 10 million and 10 billion times fainter than their parent stars. The Roman Space Telescope’s innovative coronagraph is designed to achieve a remarkable contrast sensitivity of 10-8 with inner working angles smaller than 3 λ/D. This level of precision is unprecedented in space exploration and will surpass the capabilities of previous telescopes like the Hubble Space Telescope (HST) and the James Webb Space Telescope (JWST).
With the required angular separation for the Roman coronagraph estimated between 0.13” and 0.4”, the initiative aims to vastly improve starlight suppression techniques and make strides in exoplanet detection. The High-Contrast Imaging Testbed demonstrated that starlight suppression can reach levels necessary for detecting Earth-like worlds. These developments represent a significant leap in technology, bringing us closer to the ultimate goal of identifying habitable zones where life may exist.
The operational design of the Roman Space Telescope’s LOWFS/C architecture allows for exceptional line-of-sight sensitivity, on the order of 0.4 mas. Recent testbed achievements show this technology can maintain remarkable wavefront control. Under a 14 mas vibration input, the telescope anticipates demonstrating a residual error below 0.5 mas rms, ensuring accurate measurements.
Such technological advancements are crucial for our space exploration missions, especially when considering predictions that approximately 2.7% of stars in the inner galaxy may host habitable planets. Only time and emerging technologies will reveal the true extent of life beyond our solar system, guiding scientists toward potentially promising environments for future research.
Conclusion
The study of the galactic habitable zone encapsulates a captivating frontier where the potential for life intersects with the dynamics of cosmic exploration. As we reflect on previous sections, we recognize the significance of stellar types, metallicity, and the threats posed by cosmic phenomena such as supernova explosions. These elements collectively shape the delicate fabric of habitability, revealing that while Earth remains a unique oasis within our Solar System, the search for life potential extends beyond its boundaries.
For instance, only one planet in our own Solar System lies within the habitable zone allowing liquid water, while many surrounding M stars present formidable challenges for potential life due to their intense flares and coronal mass ejections. The lifespan of these stars and their habitability dynamics often lead to rapid declines in their habitable zones as they age, underscoring the complexity of understanding where life might thrive. Moreover, advancements in planetary science, like the identification of TOI-700 d, pave the way for future discoveries that could uncover signs of life beyond our planet.
As we advance our telescopic technologies and theoretical models, the quest to unveil the intricacies of the galactic habitable zone reminds us of the vastness of our universe and the endless possibilities it holds. With ongoing cosmic exploration, our understanding of life’s origins is continually expanded, keeping the tantalizing notion of not being alone in the cosmos ever alive.