Exploring exoplanets with starshade technology.
The quest for understanding the universe has led to the discovery of exoplanets, or planets outside our solar system, revealing an array of possibilities about what lies beyond Earth. As astronomical technology advances, the need for improved observation methods has become essential.
Traditional techniques often falter due to the overwhelming brightness of host stars, masking the faint signals of these distant worlds. This is where starshade technology enters the scene, offering a revolutionary approach to direct imaging.
Approved by NASA in 2016, starshade technology aims to enhance our capability to detect exoplanets, particularly in regions known as habitable zones, where conditions might support liquid water.
With its unique design, the starshade can effectively suppress starlight, thereby allowing astronomers to discover and characterize more exoplanets than ever before.
Introduction to Exoplanets
Exoplanets, or planets outside our solar system, represent one of the most exciting frontiers in astronomy. Since the early 1990s, researchers have confirmed thousands of these celestial bodies, uncovering a fascinating array of planetary systems. Exoplanets can range from massive gas giants to smaller, potentially habitable terrestrial worlds. Each discovery propels our understanding of what lies beyond our own celestial neighborhood.
These planetary systems exhibit remarkable diversity in both composition and distance from their host stars. Some exoplanets reside in habitable zones, regions where conditions might support liquid water, a crucial ingredient for life as we understand it. The exploration of such potentially habitable worlds raises compelling questions about the existence of life elsewhere in the universe.
Understanding exoplanets plays a vital role in answering fundamental questions regarding planetary formation and evolution. In the quest to identify Earth-like planets that exist in habitable zones, ongoing research aims to characterize the atmospheres and compositions of these distant worlds. Such efforts are essential in determining which exoplanets might harbor conditions conducive to life.
This quest for knowledge has revealed that the fraction of stars likely to possess Earth-sized planets in their habitable zones is approximately 20%. With thousands of stars in our vicinity, researchers estimate that there could be numerous near-Earth-sized planets just waiting to be discovered.
The advancement of technology continues to enhance our ability to detect and analyze these distant worlds. As we refine our methods in astronomy, the prospects of finding and studying exoplanets within their habitable zones become increasingly promising.
The Need for Advanced Detection Methods
Traditional methods of exoplanet detection have provided significant insights into the universe but often depend on indirect approaches. The transit method, a popular technique, detects planets by observing the minute dimming of starlight as a planet passes in front of its host star. While effective, this method has limitations, particularly when it comes to studying the spectra and environments of exoplanets directly.
Direct imaging offers a refreshing alternative, allowing researchers to capture the light directly from an exoplanet. This approach opens a window into atmospheres, compositions, and potential habitability. Nevertheless, the faint nature of these distant worlds poses a substantial challenge. For effective exoplanet detection, starlight suppression becomes essential to isolate the weak signals emanating from these planets.
The need for advanced detection techniques is underscored by key statistics within the field. Approximately 70% of inner planets have been detected using independent component analysis methods, while about 40% of outer planets, which are significantly fainter, have also been identified. These figures highlight a pressing demand for improvements to facilitate the observation of smaller, rocky planets, especially those residing in their stars’ habitable zones.
The technologies and methodologies involved in achieving effective starlight suppression must adapt continuously. For example, a starshade designed for this purpose needs to achieve a starlight suppression of around 10^-10 to ensure success in observations. As researchers generate thousands of simulated images to refine these parameters, the development of new techniques becomes imperative to advance our understanding of exoplanets.
Condition | Inner Planets Detected (%) | Outer Planets Detected (%) | Mean Residual Brightness Error (%) |
---|---|---|---|
Using Independent Component Analysis | 70 | 40 | 10 |
The ultimate aim remains clear: refine detection methods to ensure detailed insights into exoplanetary systems and uncover the mysteries they hold.
What is Starshade Technology?
Starshade technology represents a groundbreaking innovation in the quest to explore exoplanets. This technique utilizes a specially designed occulter that effectively blocks starlight, allowing telescopes to focus on the faint light reflected from planets. The challenge of observing exoplanets has been significant; the light emitted by these distant worlds is often billions of times dimmer than that of their parent stars.
Unlike traditional coronagraph methods, which are integrated within the telescope system, starshade technology operates externally. This separation provides a much broader field of view, enhancing the ability to observe multiple celestial bodies simultaneously. The starshade has unique flower-shaped petals that create a softer light edge, resulting in reduced light wave bending, a key factor in achieving better starlight suppression.
Precision plays a crucial role in the effectiveness of the starshade. With millimeter accuracy necessary for the deployment of its petals, the technology features thrusters that allow for precise positioning, enabling it to block light from various stars as needed. This capability dramatically increases the contrast necessary for detecting fainter objects, such as rocky exoplanets.
NASA is actively working on enhancing starshade technology. Current initiatives include the development of a smaller scale starshade to verify design predictions and testing a near-full scale system under laboratory conditions. As astronomers have been discovering new exoplanets at an increasing rate, with over 200 found in the past decade, the ability of starshade technology to directly image Earth-size planets will unlock new frontiers in planetary science.
The Role of Starshade in Exoplanet Exploration
The starshade role in exoplanet exploration marks a significant advancement in astronomical techniques. By adeptly blocking starlight, starshades create clear pathways for exoplanet imaging, revealing the hidden worlds orbiting distant stars. This technology not only facilitates direct observations but also enables scientists to focus on the habitable zones surrounding stars, where conditions may be suitable for life.
One of the critical aspects of the starshade’s impact lies in its ability to enhance the characterization of atmospheres belonging to exoplanets. Scientists aim to determine the chemical composition and potential for biosignatures within these atmospheres, providing essential insights into the possibility of life beyond Earth. With improved observational capabilities, planetary systems can be examined more closely to understand their diversity and evolutionary paths.
A detailed study is underway involving two teams selected for the Starshade Exoplanet Data Challenge. This initiative focuses on quantifying the accuracy needed for background calibration necessary to detect planets as well as exozodiacal disks from synthetic images. These images incorporate various sources of background noise, such as residual starlight and solar glint, testing the limits of the technology in realistic scenarios. This rigorous imaging simulation employs the Starshade Imaging Simulation Toolkit for Exoplanet Reconnaissance (SISTER), which fully addresses astrophysical scene dimensions.
The advancements initiated by the challenge align with the goals of major upcoming missions, such as the Roman Starshade Rendezvous and HabEx missions. Expected data products will include simulated images targeting slit-prism spectroscopy and integral field spectroscopy. With these developments, astronomers will be able to explore the habitable zones of distant stars with unprecedented resolution.
The findings from the Starshade Exoplanet Data Challenge are instrumental in refining the starshade’s design and operational parameters. Documentation and synthetic images will be made available to the broader astronomy community to encourage further research and engagement in these exciting discoveries.
Data Release | Description | File Size (MB) |
---|---|---|
Data Release #1 | Selected broadband scenarios | 8.1 |
Data Release #2 | All broadband scenarios and updated instrument parameters | 87.8 |
Data Release #3 | Spectroscopy scenarios | 642.2 |
Technological Development under NASA’s ExEP
NASA’s Exoplanet Exploration Program (ExEP) plays a crucial role in advancing the development of starshade technology, focusing on overcoming significant challenges in exoplanet detection. One primary objective is to reach a Technology Readiness Level (TRL) of 5, which indicates that essential functionalities have been validated through medium-fidelity prototypes tested under relevant conditions.
A notable challenge arises from the fact that Earth-like exoplanets are incredibly faint, often measuring between 10 million and 10 billion times dimmer than the stars they orbit. The ExEP tackles this issue by refining starlight suppression techniques. These innovations must achieve a contrast ratio far exceeding the best performances currently available through ground-based observatories.
One exciting aspect in this context is the Nancy Grace Roman Telescope, designed to provide contrast sensitivity of 10^-8. This instrument will be pivotal in conducting science at wavelengths between 442 and 980 nm, employing adaptive optics and low-order wavefront sensors. Achieving the necessary level of control for light paths within the coronagraph system, specifically at sub-nanometer precision, is essential for the success of future missions.
The ExEP’s structured approach identifies three primary technology gaps for effective starshade missions:
- Starlight suppression technologies
- Formation sensing and control technology, necessary for maintaining alignment to within approximately 1 meter
- Deployment accuracy and shape stability to ensure the starshade retains its operational form
Furthermore, the starshade design utilized in the Starshade Rendezvous Mission (SRM) is projected to span 26 meters in diameter, while its successor, the Habitable Exoplanet Observatory (HabEx) mission, intends to deploy a starshade twice that size. Testing these technologies occurs in environments that simulate expected conditions in the Sun-Earth L2 halo orbit, ensuring they can withstand real mission challenges.
Starshade Exoplanet Data Challenge
The Starshade Exoplanet Data Challenge (SEDC) was designed to advance the methodologies for analyzing the images generated through starshade observations. This groundbreaking initiative centered around enhancing imaging algorithms, allowing teams in the astronomy community to refine their image-processing skills using data sets that simulated real-world scenarios.
Within the challenge, participants encountered a total of 1,440 simulated images from the Release #2 data set. The analysis was organized into three distinct sub-problems, including Background Estimation, Planet Detection, and Planet Parameter Estimation. Each area focused on maximizing the reliability of detection methods against substantial background noise.
Background estimation required evaluating eight parameters in an initial empirical model. These parameters ranged from the center position of the planet to intensity roll-off characteristics. Notably, methodologies such as Principal Component Analysis (PCA), Independent Component Analysis (ICA), and Autoencoders were scrutinized as part of the SISTER framework to improve accuracy.
The defined radius from the starshade center at which pixel data was ignored during background estimation played a crucial role. A radius of just 3 pixels created a focused environment for accurately measuring nearby signals. During this process, logarithmic loss functions were employed to better handle unmodeled signals, ultimately leading to a valuable reduction in noise levels.
Throughout this experience, the challenge fostered collaboration among participants from various institutions across Asia, Europe, and the US. Regular teleconferences facilitated fruitful discussions and enabled teams to share their insights on different atmospheric modeling scenarios, including notable components like CH4, NH3, and H2O.
The insights gained from the Starshade Exoplanet Data Challenge not only validated the starshade’s imaging capabilities but also clarified the pathway for future technological advancements in the field.
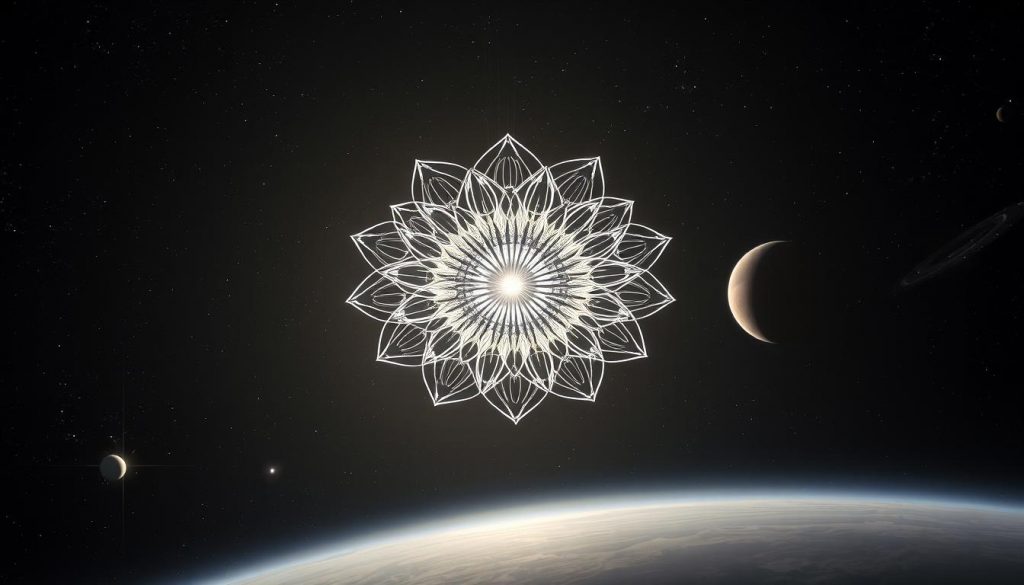
Current Status and Future Prospects of Starshade Technology
The development of starshade technology has made significant strides in recent years, marking crucial progress toward its eventual deployment. The current status of starshade missions indicates a transition from theoretical concepts to practical applications, with work being carried out under NASA’s Exoplanet Exploration Program (ExEP). Initiatives have focused on achieving technology improvements that enable more precise alignment between the starshade and the telescope.
Future prospects for starshade missions appear promising as the technology advances toward TRL5, which denotes a shift from component testing to more rigorous, functional prototype evaluations. This progress paves the way for missions that aim to observe exoplanets directly. A typical starshade mission will require two spacecraft positioned approximately 25,000 miles apart, with stringent alignment criteria of within 3 feet. Such precision translates into an extraordinary requirement of maintaining alignment within about 2 millimeters across vast distances of space.
Innovative algorithms have been developed to autonomously manage the starshade’s alignment for potentially days, greatly increasing operational feasibility. Current research has demonstrated the ability to detect misalignment down to an inch over considerable distances, showcasing the potential for precise monitoring capabilities. This leads to increased confidence in executing starshade missions that could uncover groundbreaking discoveries regarding exoplanet atmospheres and their habitability.
As we move further into the late 2020s, the anticipation surrounding these missions grows. The transition toward practical applications of these technology improvements suggests a bright future for starshade initiatives, setting the stage for transformative insights into the ever-expanding universe of exoplanet studies.
Aspect | Details |
---|---|
Distance between spacecraft | 20,000 to 40,000 kilometers |
Required alignment precision | Within 2 millimeters |
Alignment detection capability | Down to an inch over large distances |
Starshade diameter | 26 meters |
Current TRL status | Advancing from TRL 4 to TRL 5 |
Expected launch timeline | Late 2020s |
Challenges in Starshade Implementation
Implementing starshade technology brings forth significant challenges that must be meticulously addressed. One primary concern revolves around the complex deployment mechanisms that are critical for the starshade’s functionality. Ensuring accurate positioning of the starshade in relation to the telescope is vital for optimal performance during space missions. Any misalignment can severely impact the detection limits, making it difficult to observe potential exoplanets.
Environmental factors further complicate the process. The structural integrity of the starshade must withstand the rigors of space while maintaining accurate alignment. This is crucial, particularly during acceleration phases that can reach up to 0.03 g. Moreover, the starshade must recover its zero-g shape within 10 seconds or less, showcasing the engineering precision required for such missions.
Another set of challenges includes the ability to accurately model the noise environment generated by both astronomical and instrumental sources. This noise can significantly affect the imaging capabilities of starshade technology. Developing effective strategies to mitigate this noise is essential for enhancing imaging performance, which is expected to improve angular resolution by six times compared to existing telescopes.
To add to these complexities, the starshade must fit within the fairing of large rockets such as the Falcon Heavy and Starship. With a target diameter of 50 m for effective operation, managing the mass and volume while ensuring cost-effective mass management is no small feat. The design must accommodate a robust deployment structure that can be tightly packaged for transportation, applying innovative concepts like rigidizable inflatable structures or ultralight trusses.
Addressing these layers of challenges is vital for the successful integration of starshade technology in upcoming space missions. With each obstacle tackled, the vision of detecting distant exoplanets becomes more tangible, paving the way for groundbreaking discoveries in the realm of astronomy.
Collaborative Efforts in Starshade Research
Collaboration among various entities plays a crucial role in advancing starshade research. Notably, partnerships involving NASA, academic institutions, and the scientific community foster an environment primed for innovation. A standout example is the 5-member team from the University of Louisiana at Lafayette, which designed a small-scale starshade. Their project secured the third position in NASA’s Hybrid Observatory for Earth-like Exoplanets Starshade Challenge, exemplifying how teamwork can lead to significant breakthroughs.
This specific team, comprised of students from diverse fields such as physics, mechanical engineering, and geology, showcased the power of interdisciplinary collaboration. Coordinated by the Society of Physics Students, with guidance from experienced mentors like Dr. Michalis Charilaou and Dr. Yasmeen Qudsi, they utilized an inflatable truss system to create a Gas Inflated Rigid Starshade. Their impressive performance earned them $4,000 in the competition.
Overall, the starshade initiative is part of a broader ambition to enhance detection capabilities for exoplanets. The technology aims to mitigate the challenges posed by obscuring light from surrounding stars, enabling the scientific community to better observe distant worlds. With proposed starshades measuring approximately 100 meters in diameter, initiatives like the NASA Innovative Advanced Concepts (NIAC) program have dedicated significant resources, awarding $5.1 million to support 17 different researchers.
As these partnerships continue to evolve, the possibility of detecting Earth-class planets up to 7 parsecs away becomes more tangible. The collaborative spirit in starshade research is vital for advancing towards a shared goal of deeper understanding in the field of exoplanet exploration.
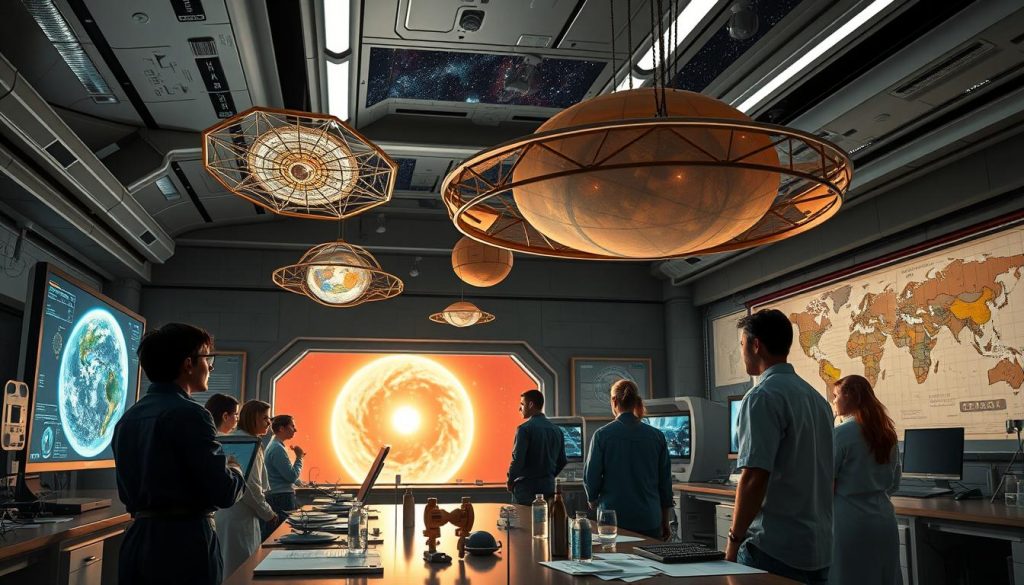
Conclusion
Starshade technology represents a revolutionary approach to exploring exoplanets, holding the promise to unveil the hidden worlds orbiting distant stars. As scientists and engineers overcome the challenges associated with starlight suppression, advanced technical capabilities are being developed to enhance our understanding of these celestial bodies. The innovative design and implementation of starshade missions, such as those proposed for the Habitable Exoplanet Observatory and the Starshade Rendezvous Mission, reflect a bright future for space science.
With the ability to block out starlight and provide high-contrast imaging, starshade technology could significantly improve our capacity to detect rocky exoplanets within habitable zones. The need for precise formation flying and alignment within just one meter underlines the sophistication required for future exploration missions. As we inch closer to achieving Technology Readiness Levels (TRL) that guarantee mission success, the scientific community stands on the brink of deeper insights into the conditions that may support life beyond Earth.
The path forward, set against a backdrop of innovative designs and collaborative efforts, inspires excitement in the pursuit of unraveling cosmic mysteries. The future of space exploration with starshade technology is filled with potential, ultimately bringing humanity closer to answering age-old questions regarding the existence of life and the multitude of exoplanets awaiting discovery. For more detailed insights into the challenges and revolutionary aspects of this technology, check out this resource.