The Enigma of Gamma-Ray Bursts and Their Connection to Galaxies
Gamma-ray bursts (GRBs) are among the universe’s most powerful and elusive cosmic phenomena. They outshine entire galaxies in mere moments. These intense bursts of gamma radiation reveal significant insights into distant galaxies and stellar evolution.
With origins confirmed in extragalactic environments, such as the notable GRB 970228, researchers have uncovered vital connections. They found that nearly 70% of GRBs may be linked to the dramatic events accompanying star formation.
The energy released during a gamma-ray burst can surpass the total output of our Sun over its entire lifespan in just a few seconds.
This staggering amount of energy and the unique characteristics of GRBs highlight the intricate relationship between these astronomical events and the broader context of galaxy formation and evolution.
As we delve deeper into understanding what drives gamma-ray bursts, we gain not only knowledge about these explosive phenomena. We also gain valuable insights into the complex dynamics of the galaxies that nurture them.
Understanding Gamma-Ray Bursts
Gamma-ray bursts (GRBs) are the most powerful explosions in the universe. They release an enormous amount of energy in seconds. This energy is as much as the Sun’s total output over its life.
GRBs are divided into two types. Long-duration GRBs last over 2 seconds and come from massive stars. These stars collapse into black holes. Short-duration GRBs, lasting less than 2 seconds, are linked to neutron star mergers.
GRBs can last from milliseconds to nearly a minute. GRB211211A is an example of a long-duration event. It raises questions about its origins, possibly linked to magnetar formation.
Kilonovae, happening during neutron star mergers, are connected to short GRBs. Long GRBs are linked to supernovae explosions. These findings highlight the importance of GRBs in astrophysics.
GRBs help create heavy elements like gold and uranium. These elements are key for planet formation and life in the universe.
Studying GRBs helps us understand the universe. By looking into their energy emissions and origins, scientists learn more about our cosmos.
Classification | Duration | Common Sources | Energy Emission |
---|---|---|---|
Long-duration GRBs | More than 2 seconds | Massive stars (30-50 solar masses) | Equivalent to the Sun’s output over a lifetime |
Short-duration GRBs | Less than 2 seconds | Neutron star mergers | Intense bursts of energy |
The Discovery of Gamma-Ray Bursts
Gamma-ray bursts (GRBs) have changed how we see the universe since the 1960s. The first one was found in 1967 by the Vela satellites. They were checking for nuclear tests, but found something unexpected.
At first, people thought these bursts came from Earth. But by the 1970s and 1980s, scientists realized they were from far-off galaxies. This discovery led to big steps forward in astrophysics.
Dr. Robert A. Miller and his team were key in figuring out GRBs’ origins. They showed these bursts come from space, not Earth. The 1990s saw better tools for studying GRBs. A big find was GRB 980703 in 1998.
GRBs are found in galaxies over 7 billion light-years away. These galaxies are very active, making stars at a rate of 20 to 500 suns per year. This shows how important GRBs are for understanding the universe.
GRBs are divided into short and long bursts. Short ones last under 2 seconds, and long ones can last for hours. Short bursts come from neutron star mergers, and long ones from massive star collapses.
Studying GRBs keeps giving us new insights into the universe and black holes. Future upgrades to the VLA will help find more GRB host galaxies. This will help us understand how galaxies and GRBs evolve together.
Aspect | Short-Duration GRBs | Long-Duration GRBs |
---|---|---|
Duration | Less than 2 seconds | Several minutes to hours |
Cause | Merging neutron stars | Collapse of massive stars |
Detection Range | Typically less than billions of light-years | Can be detected from billions of light-years away |
Energy Released | Equivalent to several suns’ mass | Varied, significant energy output |
The Science Behind Gamma-Ray Bursts
Gamma-Ray Bursts (GRBs) are caused by complex astrophysical processes. They show us the universe’s most violent events. Long-duration GRBs come from massive stars collapsing, forming black holes and powerful jets.
These jets move almost as fast as light, releasing huge amounts of energy. This makes GRBs rare and amazing.
Short-duration GRBs happen when compact stellar objects, like neutron stars, merge. This violent event also creates high-energy radiation. It shows the same destructive power as long-duration GRBs.
GRBs occur in about 1 out of every 10,000 massive stars. Only stars with 30 to 100 times the Sun’s mass can cause them. A GRB was found 3,000 light-years from a galaxy’s star clusters, showing their link to stars.
In massive stellar superclusters, intense star interactions can push stars fast. This can lead to a GRB. GRBs are rare, but nearly one is seen every day. Research has grown, increasing GRB observations from 10-20 a year to over 300 today.
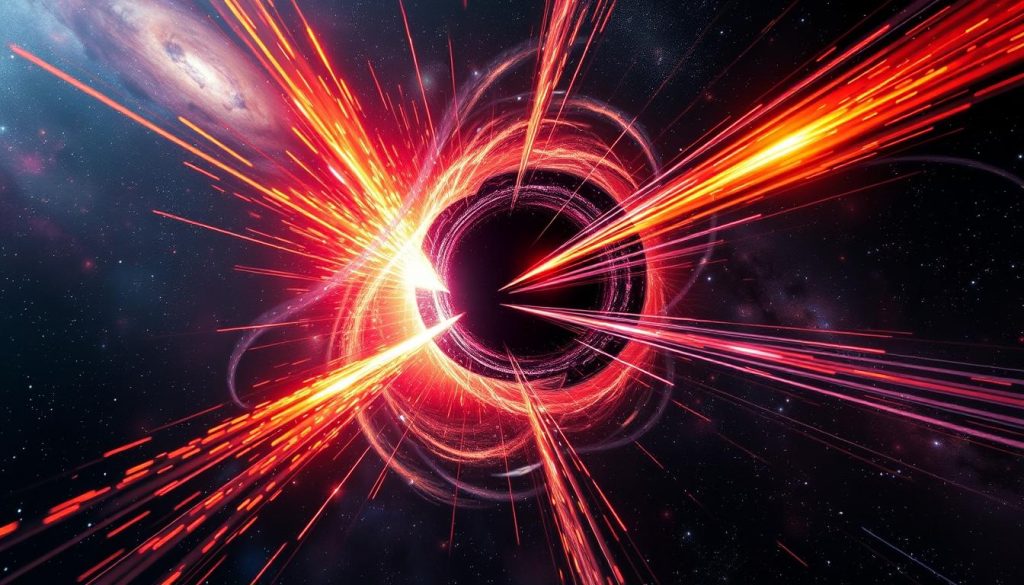
GRBs have varied durations, from 30 milliseconds to over 1,000 seconds. This makes them highly variable. Some bursts have delayed emissions, showing their complexity.
A notable GRB lasted 180 seconds, with more emissions for over 5,700 seconds after. This shows their complexity.
Characteristic | Details |
---|---|
Occurrence Rate | Approximately 1 in 10,000 massive stars |
Star Mass Range | 30 to 100 times that of the Sun |
Daily Observations | About 1 GRB per day |
Annual Observations (Current) | Over 300 GRBs |
Observation Duration | From 30 milliseconds up to 1,000 seconds |
Delayed Emission | Extends to over 5,700 seconds |
These data help researchers understand GRBs better. As interest grows, we’ll learn more about these cosmic wonders.
Galaxies and Their Connection to Gamma-Ray Bursts
Gamma-ray bursts (GRBs) and their host galaxies offer key insights into galaxy formation and cosmic evolution. GRBs often signal intense star formation, mainly in galaxies’ star-forming zones. By detecting GRBs, astronomers can trace back star formation activities and understand galaxy lifecycles.
For example, GRB 060206’s redshift of z = 4.04795 ± 0.00020 reveals the universe’s past. It has a high metallicity of [S/H] = -0.84 ± 0.10, one of the highest at such redshifts. This shows the universe’s chemical evolution and how GRBs mark galaxy evolution stages.
The presence of neutral hydrogen around GRB host galaxies is significant. A column density of log N(H i) = 20.85 ± 0.10 indicates a lot of gaseous material. This environment is perfect for forming new stars and galaxies. Also, detecting molecular hydrogen with log N(H2) ∼ 17.0 shows a large molecular fraction. These findings connect GRB host galaxies with galaxy formation processes.
GRB 060206’s analysis shows its complexity. With a T90 duration of 7 ± 2 seconds, it highlights the dynamic nature of these events. Comparing column densities for sulfur and silicon ions, we see the material composition in star-forming regions.
The link between GRBs and their host galaxies helps us understand galaxy formation and cosmic evolution. Each GRB we study brings us closer to understanding the universe’s structure and the dance of stars.
Parameter | Observation |
---|---|
Redshift of GRB 060206 | z = 4.04795 ± 0.00020 |
Metallicity | [S/H] = -0.84 ± 0.10 |
Neutral Hydrogen Column Density | log N(H i) = 20.85 ± 0.10 |
Molecular Hydrogen Column Density | log N(H2) ∼ 17.0 |
T90 Duration | 7 ± 2 seconds |
Occurrence of High Metallicity Measurement | One of the highest at z ∼ 4 |
[Si ii*/S iii] Ratio | -1.15 and -0.34 for different components |
The Enigma of Gamma-Ray Bursts
Gamma-ray bursts (GRBs) are a big mystery for astrophysicists. They are among the most powerful explosions in the universe. Yet, we still don’t fully understand how they work or what causes them.
The discovery of GRB221009A has changed how we think about GRBs. It showed an energy peak of 10 Mega-Electron-Volts (MeV), much higher than before. This shows that GRBs are more complex than we thought, and we need to look at them in a new way.
Scientists think that the energy in GRBs comes from the collision of electrons and positrons. This collision creates gamma rays with a specific energy. This idea might help us understand GRBs better, especially since old theories didn’t work well.
Studies show that GRBs are happening more often. The rate of star formation in their host galaxies is about 500 solar masses per year. This suggests a strong connection between GRBs and their surroundings, which is helping scientists learn more about them.
The Role of Supernovae and Hypernovae
Supernovae and hypernovae are key moments in massive stars’ lives. They affect gamma-ray emission in big ways. Type II supernovae lead to long-duration gamma-ray bursts (GRBs). These happen when stars bigger than eight suns run out of fuel and collapse.
Hypernovae, with ten times the energy of a normal supernova, are linked to GRBs with super-strong gamma-ray emission. Events like ASASSN-15lh show how powerful these can be. It was the most energetic supernova ever seen, shining 200 times brighter than usual.
Studying supernovae, hypernovae, and GRBs helps us understand stars better. Some think hypernovae might cause certain GRBs. This shows how these events are connected.
These events can outshine whole galaxies at their peak. They release energy that helps create new stars and planets. This cycle links these cosmic events to the universe’s growth.
Event Type | Energy Output | Mass Threshold |
---|---|---|
Standard Supernova | Typical output | 8 solar masses |
Hypernova | 10 times standard supernova | ≥ 20 solar masses |
ASASSN-15lh | 200 times typical supernova | Not specified |
For more on supernovae and gamma-ray bursts, check out this in-depth study. Learning about these events helps us understand stars and the universe’s history.
Observational Techniques for Studying Gamma-Ray Bursts
Gamma-ray bursts (GRBs) have fascinated scientists for over 40 years. Since their discovery in the late 1960s, many methods have been used to study them. Space telescopes like NASA’s Swift satellite help by quickly finding GRBs and their afterglows.
Both ground-based and space telescopes, like the Hubble Space Telescope and the Keck Observatory, help in real-time studies. They look at GRBs across different wavelengths. This is key to understanding their properties and behaviors.
Recent efforts aim to improve very high energy gamma-ray observations. The goal is to increase sensitivity by over an order of magnitude in the next decade. GRBs are divided into long and short types. Long GRBs come from massive star deaths, while short GRBs are from mergers of compact objects.
Observations show that GRB afterglows can vary greatly in brightness. Some are very bright, while others are hard to detect. High redshifts, dust in host galaxies, and GRBs in low-density areas play roles in these differences. The Panchromatic Robotic Optical Monitoring and Polarimetry Telescopes (PROMPT) have improved finding dim and dark GRBs.
PROMPT can start observing within seconds of a GRB event. This quick response helps in understanding GRBs better. It also helps in figuring out why some GRBs are bright and others are not.
The Significance of Gamma-Ray Bursts in Astrophysics
Gamma-ray bursts (GRBs) are key in astrophysics, boosting their importance in many areas of study. They are now detected more often, with over 300 found each year. This is a big jump from the 10 to 20 found in the early days after they were first spotted.
These cosmic events seem to happen randomly, about once every day. Their unpredictable nature makes them fascinating to study. GRBs can last anywhere from 30 milliseconds to over 1000 seconds, with the longest one lasting 5700 seconds in February 1994.
This wide range in duration makes them even more interesting to scientists. They want to know what causes these bursts. Most of the energy from GRBs is above 50 keV, showing how bright and energetic they can be.
There have been over 2,000 papers on GRBs in the last 25 years. This shows how much scientists are interested in them. The BATSE experiment on NASA’s Compton Gamma Ray Observatory has been key in understanding GRBs since April 1991.
GRBs are like natural labs for studying high-energy physics. They help us learn about star formation, dark matter, and dark energy. This knowledge helps us improve our understanding of the universe.
Statistic | Value |
---|---|
Annual Detection Rate | Over 300 |
Average Occurrence | Approximately once per day |
Duration Range | 30 ms to 5700 s |
Peak Energy | Above 50 keV |
Published Papers | Over 2000 |
Notable Experiment | BATSE (NASA’s Compton Gamma Ray Observatory) |
Known Redshifts of XRFs | Range from 0.03342 to 2.658 |
The Future of Gamma-Ray Burst Research
The field of gamma-ray burst research is on the verge of a big change. This change comes from upcoming missions and new ways of studying these events. The James Webb Space Telescope and the Gamma-ray Observatory are just a few examples of what’s coming.
Researchers are excited to learn more about these powerful events. They’re looking into how the energy of gamma-ray bursts changes over time. This includes how the bursts start off strong and then fade, and how the energy changes as they do.
New models are being developed to better understand gamma-ray bursts. These models will help us see how bursts fit into the bigger picture of the universe. By studying the light from these bursts, scientists are learning more about their nature.
The Gamma-Ray Astronomy Program Working Group is also playing a big role. They focus on high-energy astrophysics, which includes gamma-ray bursts. Even missions from years ago, like Swift, are still helping us learn more today.
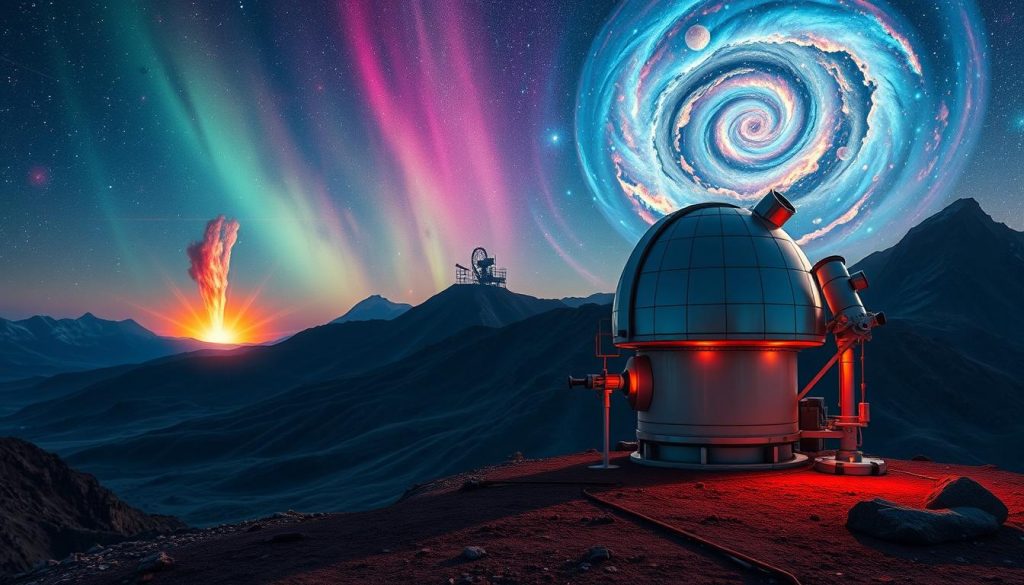
The search for knowledge in gamma-ray burst research will keep going strong. Scientists are diving into the details of how these bursts work. They’re looking at things like magnetic fields and the flow of matter. This will help us understand more about these powerful events and their place in the universe.
Challenges in Understanding Gamma-Ray Bursts
Gamma-ray bursts (GRBs) are tough for scientists to study. Observational limitations are a big problem. These cosmic events are rare and short-lived. This makes it hard to get all the data needed to fully understand them.
There are also theoretical challenges. Scientists have to fit together different models of how GRBs form and what happens after they occur. For example, some bursts, like GRB211211A, last almost a minute. This surprises scientists and makes it harder to match observations with simulations.
Using data from gravitational waves is key to solving these problems. However, detectors like LIGO, Virgo, and KAGRA missed GRB211211A. New efforts in 2023 aim to catch long-duration GRBs and their gravitational waves. This could be a big step forward.
In summary, scientists face many challenges in studying GRBs. They need new ways and better strategies to overcome these theoretical challenges. This will help us understand the universe better. For more on recent discoveries, check out this important source.
Type of Challenge | Description |
---|---|
Observational Limitations | Rarity and transient nature of GRBs hinder data collection. |
Theoretical Challenges | Need for harmony among various GRB formation models. |
Data Integration | Incorporating gravitational wave data remains a key hurdle. |
Conclusion
Gamma-ray bursts (GRBs) are fascinating in astrophysics, giving us a peek into the universe’s dramatic events. They show how GRBs are linked to galaxies, highlighting their role in understanding the cosmos. As scientists keep studying, we’ll learn more about the universe through GRBs.
The search for GRB secrets is just starting. With new tech, researchers will explore these bursts more. Each finding will help us understand the universe better, showing us more about GRBs and their galaxies.
GRBs will keep being a major focus in studying the cosmos. They offer key insights that could change how we see the universe. The mystery of GRBs sparks our curiosity, showing us the wonders waiting to be found in space.